Magnetic resonance imaging
![]() | This article is written like a a personal reflection or opinion essay that states the Wikipedia editor's particular feelings about a topic, rather than the opinions of experts. (February 2015) |
Template:Infobox diagnostic Magnetic resonance imaging (MRI), nuclear magnetic resonance imaging (NMRI), or magnetic resonance tomography (MRT) is a medical imaging technique used in radiology to investigate the anatomy and physiology of the body in both health and disease. MRI scanners use strong magnetic fields and radio waves to form images of the body. The technique is widely used in hospitals for medical diagnosis, staging of disease and for follow-up without exposure to ionizing radiation.
Introduction
[edit]MRI has a wide range of applications in medical diagnosis and there are estimated to be over 25,000 scanners in use worldwide.[1] MRI has an impact on diagnosis and treatment in many specialties although the effect on improved health outcomes is uncertain.[2] Since MRI does not use any ionizing radiation its use is recommended in preference to CT when either modality could yield the same information.[3] MRI is in general a safe technique but the number of incidents causing patient harm have risen.[4] Contraindications to MRI include most cochlear implants and cardiac pacemakers, shrapnel and metallic foreign bodies in the orbits. The safety of MRI during the first trimester of pregnancy is uncertain, but it may be preferable to alternative options.[5] The sustained increase in demand for MRI within the healthcare industry has led to concerns about cost effectiveness and overdiagnosis.[6][7]
Neuroimaging
[edit]
MRI is the investigative tool of choice for neurological cancers as it is more sensitive than CT for small tumors and offers better visualization of the posterior fossa. The contrast provided between grey and white matter makes it the optimal choice for many conditions of the central nervous system including demyelinating diseases, dementia, cerebrovascular disease, infectious diseases and epilepsy.[8] MRI is also used in MRI-guided stereotactic surgery and radiosurgery for treatment of intracranial tumors, arteriovenous malformations and other surgically treatable conditions using a device known as the N-localizer.[9][10][11][12]
Cardiovascular
[edit]
Cardiac MRI is complementary to other imaging techniques, such as echocardiography, cardiac CT and nuclear medicine. Its applications include assessment of myocardial ischemia and viability, cardiomyopathies, myocarditis, iron overload, vascular diseases and congenital heart disease.[13]
Musculoskeletal
[edit]Applications in the musculoskeletal system include spinal imaging, assessment of joint disease and soft tissue tumors.[14]
Liver and gastrointestinal MRI
[edit]Hepatobiliary MR is used to detect and characterize lesions of the liver, pancreas and bile ducts. Focal or diffuse disorders of the liver may be evaluated using diffusion-weighted, opposed-phase imaging and dynamic contrast enhancement sequences. Extracellular contrast agents are widely used in liver MRI and newer hepatobiliary contrast agents also provide the opportunity to perform functional biliary imaging. Anatomical imaging of the bile ducts is achieved by using a heavily T2-weighted sequence in magnetic resonance cholangiopancreatography (MRCP). Functional imaging of the pancreas is performed following administration of secretin. MR enterography provides non-invasive assessment of inflammatory bowel disease and small bowel tumors. MR-colonography can play a role in the detection of large polyps in patients at increased risk of colorectal cancer.[15][16][17][18]
Functional MRI
[edit]Functional MRI (fMRI) is used to understand how different parts of the brain respond to external stimuli. Blood oxygenation level dependent (BOLD) fMRI measures the hemodynamic response to transient neural activity resulting from a change in the ratio of oxyhemoglobin and deoxyhemoglobin. Statistical methods are used to construct a 3D parametric map of the brain indicating those regions of the cortex which demonstrate a significant change in activity in response to the task. FMRI has applications in behavioral and cognitive research as well as in planning neurosurgery of eloquent brain areas.[19][20]
Oncology
[edit]MRI is the investigation of choice in the preoperative staging of rectal and prostate cancer, and has a role in the diagnosis, staging, and follow-up of other tumors.[21]
How MRI works
[edit]To perform a study, the patient is positioned within an MRI scanner which forms a strong magnetic field around the area to be imaged. In most medical applications, protons (hydrogen atoms) in tissues containing water molecules are used to create a signal that is processed to form an image of the body. First, energy from an oscillating magnetic field is temporarily applied to the patient at the appropriate resonant frequency. The excited hydrogen atoms emit a radio frequency signal which is measured by a receiver coil. The radio signal can be made to encode position information by varying the main magnetic field using gradient coils. As these coils are rapidly switched on and off they create the characteristic repetitive noises of an MRI scan. The contrast between different tissues is determined by the rate at which excited atoms return to the equilibrium state. Exogenous contrast agents may be given intravenously, orally or intra-articularly.[22]
MRI requires a magnetic field that is both strong and uniform. The field strength of the magnet is measured in tesla – and while the majority of systems operate at 1.5T, commercial systems are available between 0.2T–7T. Most clinical magnets are superconducting which requires liquid helium. The lower field strengths can be achieved with permanent magnets, which are often used in "open" MRI scanners for claustrophobic patients.[23]
Contrast in MRI
[edit]

Image contrast may be weighted to demonstrate different anatomical structures or pathologies. Each tissue returns to its equilibrium state after excitation by the independent processes of T1 (spin-lattice) and T2 (spin-spin) relaxation.
To create a T1-weighted image more magnetization is allowed to recover before measuring the MR signal by changing the repetition time (TR). This image weighting is useful for assessing the cerebral cortex, identifying fatty tissue, characterizing focal liver lesions and for post-contrast imaging.
To create a T2-weighted image more magnetization is allowed to decay before measuring the MR signal by changing the echo time (TE). This image weighting is useful for detecting edema, revealing white matter lesions and assessing zonal anatomy in the prostate and uterus.
History
[edit]Magnetic resonance imaging was invented by Paul C. Lauterbur in September 1971; he published the theory behind it in March 1973.[24][25] The factors leading to image contrast (differences in tissue relaxation time values) had been described nearly 20 years earlier by Erik Odeblad (physician and scientist).[26]
In 1952, Herman Carr produced a one-dimensional NMR spectrum as reported in his Harvard PhD thesis.[27][28][29] In the Soviet Union, Vladislav Ivanov filed (in 1960) a document with the USSR State Committee for Inventions and Discovery at Leningrad for a Magnetic Resonance Imaging device,[30] although this was not approved until the 1970s.[31]

In a 1971 paper in the journal Science,[32] Raymond Damadian, an American physician and professor at the Downstate Medical Center State University of New York (SUNY), reported that tumors and normal tissue can be distinguished in vivo by nuclear magnetic resonance ("NMR"). He suggested that these differences could be used to diagnose cancer, though later research would find that these differences, while real, are too variable for diagnostic purposes. Damadian's initial methods were flawed for practical use,[33] relying on a point-by-point scan of the entire body and using relaxation rates, which turned out not to be an effective indicator of cancerous tissue.[34] While researching the analytical properties of magnetic resonance, Damadian created a hypothetical magnetic resonance cancer-detecting machine in 1972. He filed the first patent for such a machine, U.S. patent #3,789,832 on March 17, 1972, which was later issued to him on February 5, 1974.[35]
The National Science Foundation notes "The patent included the idea of using NMR to 'scan' the human body to locate cancerous tissue."[36] However, it did not describe a method for generating pictures from such a scan or precisely how such a scan might be done.[37][38] Meanwhile, Paul Lauterbur at Stony Brook University expanded on Carr's technique and developed a way to generate the first MRI images, in 2D and 3D, using gradients. In 1973, Lauterbur published the first nuclear magnetic resonance image[24][39] and the first cross-sectional image of a living mouse in January 1974.[40] In the late 1970s, Peter Mansfield, a physicist and professor at the University of Nottingham, England, developed a mathematical technique that would allow scans to take seconds rather than hours and produce clearer images than Lauterbur had. Damadian, along with Larry Minkoff and Michael Goldsmith, performed the first MRI body scan of a human being on July 3, 1977,[41][42] studies which they published in 1977.[43][44] In 1979, Richard S. Likes filed a patent on k-space *4,307,343.
During the 1970s a team led by Scottish professor John Mallard built the first full body MRI scanner at the University of Aberdeen.[45] On 28 August 1980 they used this machine to obtain the first clinically useful image of a patient’s internal tissues using Magnetic Resonance Imaging (MRI), which identified a primary tumour in the patient's chest, an abnormal liver, and secondary cancer in his bones.[46] This machine was later used at St Bartholomew’s Hospital, in London, from 1983 to 1993. Mallard and his team are credited for technological advances that led to the widespread introduction of MRI.[47]
In 1980 Paul Bottomley joined the GE Research Center in Schenectady, NY, and his team ordered the highest field-strength magnet then available — a 1.5T system — and built the first high-field and overcame problems of coil design, RF penetration and signal-to-noise ratio to build the first whole-body MRI/MRS scanner.[48] The results translated into the highly successful 1.5T MRI product-line, with over 20,000 systems in use today. In 1982, Bottomley performed the first localized MRS in the human heart and brain. After starting a collaboration on heart applications with Robert Weiss at Johns Hopkins, Bottomley returned to the university in 1994 as Russell Morgan Professor and director of the MR Research Division.[49] Although MRI is most commonly performed at 1.5 T, higher fields such as 3T are gaining more popularity because of their increased sensitivity and resolution. In research laboratories, human studies have been performed at up to 9.4 T[50] and animal studies have been performed at up to 21.1T.[51]
2003 Nobel Prize
[edit]Reflecting the fundamental importance and applicability of MRI in medicine, Paul Lauterbur of the University of Illinois at Urbana-Champaign and Sir Peter Mansfield of the University of Nottingham were awarded the 2003 Nobel Prize in Physiology or Medicine for their "discoveries concerning magnetic resonance imaging". The Nobel citation acknowledged Lauterbur's insight of using magnetic field gradients to determine spatial localization, a discovery that allowed rapid acquisition of 2D images. Mansfield was credited with introducing the mathematical formalism and developing techniques for efficient gradient utilization and fast imaging. The actual research that won the prize was done almost 30 years before while Paul Lauterbur was a professor in the Department of Chemistry at Stony Brook University in New York.[24]
Safety of MRI
[edit]Implants
[edit]All patients are reviewed for contraindications prior to MRI scanning. Medical devices and implants are categorized as MR Safe, MR Conditional or MR Unsafe: [52]
- MR-Safe — The device or implant is completely non-magnetic, non-electrically conductive, and non-RF reactive, eliminating all of the primary potential threats during an MRI procedure.
- MR-Conditional — A device or implant that may contain magnetic, electrically conductive or RF-reactive components that is safe for operations in proximity to the MRI, provided the conditions for safe operation are defined and observed (such as 'tested safe to 1.5 teslas' or 'safe in magnetic fields below 500 gauss in strength').
- MR-Unsafe — Objects that are significantly ferromagnetic and pose a clear and direct threat to persons and equipment within the magnet room.
The MRI environment may cause harm in patients with MR-Unsafe devices such as cochlear implants and most permanent pacemakers. Several deaths have been reported in patients with pacemakers who have undergone MRI scanning without appropriate precautions.[53] Many implants can be safely scanned if the appropriate conditions are adhered to and these are available online (see www.MRIsafety.com). MR Conditional pacemakers are increasingly available for selected patients. [54]
Ferromagnetic foreign bodies such as shell fragments, or metallic implants such as surgical prostheses and ferromagnetic aneurysm clips are also potential risks. Interaction of the magnetic and radio frequency fields with such objects can lead to heating or torque of the object during an MRI.[55]
Titanium and its alloys are safe from attraction and torque forces produced by the magnetic field, though there may be some risks associated with Lenz effect forces acting on titanium implants in sensitive areas within the subject, such as stapes implants in the inner ear.
Projectile risk
[edit]The very high strength of the magnetic field can cause projectile effect (or "missile-effect") accidents, where ferromagnetic objects are attracted to the center of the magnet. Pennsylvania reported 27 cases of objects becoming projectiles in the MRI environment between 2004 and 2008.[56] There have been incidents of injury and death.[57][58] In one tragic case, a 6-year-old boy died after an MRI exam, after a metal oxygen tank was pulled across the room and crushed the child's head.[59]
To reduce the risk of projectile accidents, ferromagnetic objects and devices are typically prohibited in the proximity of the MRI scanner and patients undergoing MRI examinations are required to remove all metallic objects, often by changing into a gown or scrubs, and ferromagnetic detection devices are used at some sites.[60][61]
EEG Cup Electrodes
[edit]EEG (electroencephalography) cup electrodes or are categorized as medical accessories and the same MR Safe, MR Conditional and MR Unsafe terminology applies. With the growth of the use of MR technology, the U.S. Food & Drug Administration [FDA] recognized the need for a consensus on standards of practice, and the FDA sought out ASTM International [ASTM] to achieve them. Working with key stakeholders, Committee F04[62] of ASTM developed F2503, Standard Practice for Marking Medical Devices and Other Items for Safety in the Magnetic Resonance Environment.[63]
Genotoxic effects
[edit]There is no proven risk of biological harm from even very powerful static magnetic fields.[64][65] However, genotoxic (i.e., potentially carcinogenic) effects of MRI scanning have been demonstrated in vivo and in vitro,[66][67][68][69] leading a recent review to recommend "a need for further studies and prudent use in order to avoid unnecessary examinations, according to the precautionary principle".[65] In a comparison of genotoxic effects of MRI compared with those of CT scans, Knuuti et al. reported that even though the DNA damage detected after MRI was at a level comparable to that produced by scans using ionizing radiation (low-dose coronary CT angiography, nuclear imaging, and X-ray angiography), differences in the mechanism by which this damage takes place suggests that the cancer risk of MRI, if any, is unknown.[70]
Peripheral nerve stimulation (PNS)
[edit]The rapid switching on and off of the magnetic field gradients is capable of causing nerve stimulation. Volunteers report a twitching sensation when exposed to rapidly switched fields, particularly in their extremities.[71][72] The reason the peripheral nerves are stimulated is that the changing field increases with distance from the center of the gradient coils (which more or less coincides with the center of the magnet).[73] Although PNS was not a problem for the slow, weak gradients used in the early days of MRI, the strong, rapidly switched gradients used in techniques such as EPI, fMRI, diffusion MRI, etc. are capable of inducing PNS. American and European regulatory agencies insist that manufacturers stay below specified dB/dt limits (dB/dt is the change in magnetic field strength per unit time) or else prove that no PNS is induced for any imaging sequence. As a result of dB/dt limitation, commercial MRI systems cannot use the full rated power of their gradient amplifiers.
Heating caused by absorption of radio waves
[edit]Every MRI scanner has a powerful radio transmitter to generate the electromagnetic field which excites the spins. If the body absorbs the energy, heating occurs. For this reason, the transmitter rate at which energy is absorbed by the body has to be limited (see Specific absorption rate). It has been argued ([5], [6]) that tattoos made with iron containing dyes can lead to burns on the subject's body.
Acoustic noise
[edit]Switching of field gradients causes a change in the Lorentz force experienced by the gradient coils, producing minute expansions and contractions of the coil itself. As the switching is typically in the audible frequency range, the resulting vibration produces loud noises (clicking or beeping). This is most marked with high-field machines[74] and rapid-imaging techniques in which sound pressure levels can reach 120 dB(A) (equivalent to a jet engine at take-off),[75] and therefore appropriate ear protection is essential for anyone inside the MRI scanner room during the examination.[76]
Cryogens
[edit]As described in Physics of Magnetic Resonance Imaging, many MRI scanners rely on cryogenic liquids to enable the superconducting capabilities of the electromagnetic coils within. Though the cryogenic liquids used are non-toxic, their physical properties present specific hazards.[77]
An unintentional shut-down of a superconducting electromagnet, an event known as "quench", involves the rapid boiling of liquid helium from the device. If the rapidly expanding helium cannot be dissipated through an external vent, sometimes referred to as a 'quench pipe', it may be released into the scanner room where it may cause displacement of the oxygen and present a risk of asphyxiation.[78]
Oxygen deficiency monitors are usually used as a safety precaution. Liquid helium, the most commonly used cryogen in MRI, undergoes near explosive expansion as it changes from a liquid to gaseous state. The use of an oxygen monitor is important to ensure that oxygen levels are safe for patient/physicians. Rooms built for superconducting MRI equipment should be equipped with pressure relief mechanisms[79] and an exhaust fan, in addition to the required quench pipe.
Because a quench results in rapid loss of cryogens from the magnet, recommissioning the magnet is expensive and time-consuming. Spontaneous quenches are uncommon, but a quench may also be triggered by an equipment malfunction, an improper cryogen fill technique, contaminants inside the cryostat, or extreme magnetic or vibrational disturbances.[80][81]
Pregnancy
[edit]No effects of MRI on the fetus have been demonstrated.[82] In particular, MRI avoids the use of ionizing radiation, to which the fetus is particularly sensitive. However, as a precaution, current guidelines recommend that pregnant women undergo MRI only when essential. This is particularly the case during the first trimester of pregnancy, as organogenesis takes place during this period. The concerns in pregnancy are the same as for MRI in general, but the fetus may be more sensitive to the effects—particularly to heating and to noise. The use of gadolinium-based contrast media in pregnancy is an off-label indication and may only be administered in the lowest dose required to provide essential diagnostic information.[83]
Despite these concerns, MRI is rapidly growing in importance as a way of diagnosing and monitoring congenital defects of the fetus because it can provide more diagnostic information than ultrasound and it lacks the ionizing radiation of CT. MRI without contrast agents is the imaging mode of choice for pre-surgical, in-utero diagnosis and evaluation of fetal tumors, primarily teratomas, facilitating open fetal surgery, other fetal interventions, and planning for procedures (such as the EXIT procedure) to safely deliver and treat babies whose defects would otherwise be fatal.[citation needed]
Claustrophobia and discomfort
[edit]Although painless, MRI scans can be unpleasant for those who are claustrophobic or otherwise uncomfortable with the imaging device surrounding them. Older closed bore MRI systems have a fairly long tube or tunnel. The part of the body being imaged must lie at the center of the magnet, which is at the absolute center of the tunnel. Because scan times on these older scanners may be long (occasionally up to 40 minutes for the entire procedure), people with even mild claustrophobia are sometimes unable to tolerate an MRI scan without management. Some modern scanners have larger bores (up to 70 cm) and scan times are shorter. A 1.5 T wide short bore scanner increases the examination success rate in patients with claustrophobia and substantially reduces the need for anesthesia-assisted MRI examinations even when claustrophobia is severe.[84]
Alternative scanner designs, such as open or upright systems, can also be helpful where these are available. Though open scanners have increased in popularity, they produce inferior scan quality because they operate at lower magnetic fields than closed scanners. However, commercial 1.5 tesla open systems have recently become available, providing much better image quality than previous lower field strength open models.[85]
Mirror glasses can be used to help create the illusion of openness. The mirrors are angled at 45 degrees, allowing the patient to look down their body and out the end of the imaging area. The appearance is of an open tube pointing upwards (as seen when lying in the imaging area). Even though one can see around the glasses and the proximity of the device is very evident, this illusion is quite persuasive and relieves the claustrophobic feeling.
For babies and other young children, chemical sedation or general anesthesia are the norm, as these subjects cannot be expected or instructed to hold still during the scanning session. Children are also frequently sedated because they are frightened by the unfamiliar procedure and the loud noises. To reduce anxiety, some hospitals have specially designed child-friendly approaches that pretend the MRI machine is a spaceship or other fun experience.[86]
Obese patients and pregnant women may find the MRI machine to be a tight fit. Pregnant women in the third trimester may also have difficulty lying on their backs for an hour or more without moving.
MRI versus CT
[edit]MRI and computed tomography (CT) are complementary imaging technologies and each has advantages and limitations for particular applications. CT is more widely used than MRI in OECD countries with a mean of 132 vs 46 exams per 1000 population performed respectively.[87] A concern is the potential for CT to contribute to radiation-induced cancer and in 2007 it was estimated that 0.4% of current cancers in the United States were due to CTs performed in the past, and that in the future this figure may rise to 1.5–2% based on historical rates of CT usage.[88] An Australian study found that one in every 1800 CT scans was associated with an excess cancer.[89] An advantage of MRI is that no ionizing radiation is used and so it is recommended over CT when either approach could yield the same diagnostic information.[3] However, although the cost of MRI has fallen, making it more competitive with CT, there are not many common imaging scenarios in which MRI can simply replace CT, although this substitution has been suggested for the imaging of liver disease.[90] The effect of low doses of radiation on carcinogenesis are also disputed.[91] Although MRI is associated with biological effects, these have not been proven to cause measurable harm.[92] In a comparison of possible genotoxic effects of MRI compared with those of CT scans, Knuuti et al. noted that although previous studies have demonstrated DNA damage associated with MRI, "the long-term biological and clinical significance of DNA double-strand breaks induced by MRI remains unknown".[70]
Iodinated contrast medium is routinely used in CT and the main adverse events are anaphylactoid reactions and nephrotoxicity.[93] Commonly used MRI contrast agents have a good safety profile but linear non-ionic agents in particular have been implicated in nephrogenic systemic fibrosis in patients with severely impaired renal function.[94]
MRI is contraindicated in the presence of MR-unsafe implants, and although these patients may be imaged with CT, beam hardening artefact from metallic devices, such as pacemakers and implantable cardioverter-defibrillators, may also affect image quality.[95] MRI is a longer investigation than CT and an exam may take between 20 - 40 mins depending on complexity.[96]
Guidance
[edit]Safety issues, including the potential for biostimulation device interference, movement of ferromagnetic bodies, and incidental localized heating, have been addressed in the American College of Radiology's White Paper on MR Safety, which was originally published in 2002 and expanded in 2004. The ACR White Paper on MR Safety has been rewritten and was released early in 2007 under the new title ACR Guidance Document for Safe MR Practices.
In December 2007, the Medicines and Healthcare Products Regulatory Agency (MHRA), a UK healthcare regulatory body, issued their Safety Guidelines for Magnetic Resonance Imaging Equipment in Clinical Use.
In February 2008, the Joint Commission, a US healthcare accrediting organization, issued a Sentinel Event Alert #38, their highest patient safety advisory, on MRI safety issues.
In July 2008, the United States Veterans Administration, a federal governmental agency serving the healthcare needs of former military personnel, issued a substantial revision to their MRI Design Guide,[97] which includes physical and facility safety considerations.
The European Directive on Electromagnetic Fields
[edit]This Directive (2013/35/EU - electromagnetic fields) [98] covers all known direct biophysical effects and indirect effects caused by electromagnetic fields within the EU and repealed the 2004/40/EC directive. The deadline for implementation of the new directive is 1 July 2016. Article 10 of the directive sets out the scope of the derogation for MRI, stating that the exposure limits may be exceeded during "the installation, testing, use, development, maintenance of or research related to magnetic resonance imaging (MRI) equipment for patients in the health sector, provided that certain conditions are met." Uncertainties remain regarding the scope and conditions of this derogation.[99]
Contrast agents
[edit]The most commonly used intravenous contrast agents are based on chelates of gadolinium.[100] In general, these agents have proved safer than the iodinated contrast agents used in X-ray radiography or CT. Anaphylactoid reactions are rare, occurring in approx. 0.03–0.1%.[101] Of particular interest is the lower incidence of nephrotoxicity, compared with iodinated agents, when given at usual doses—this has made contrast-enhanced MRI scanning an option for patients with renal impairment, who would otherwise not be able to undergo contrast-enhanced CT.[102]
Although gadolinium agents have proved useful for patients with renal impairment, in patients with severe renal failure requiring dialysis there is a risk of a rare but serious illness, nephrogenic systemic fibrosis, which may be linked to the use of certain gadolinium-containing agents. The most frequently linked is gadodiamide, but other agents have been linked too.[103] Although a causal link has not been definitively established, current guidelines in the United States are that dialysis patients should only receive gadolinium agents where essential, and that dialysis should be performed as soon as possible after the scan to remove the agent from the body promptly.[104][105] In Europe, where more gadolinium-containing agents are available, a classification of agents according to potential risks has been released.[106][107] Recently, a new contrast agent named gadoxetate, brand name Eovist (US) or Primovist (EU), was approved for diagnostic use: this has the theoretical benefit of a dual excretion path.[108]
Health economics
[edit]In the UK, the price of a clinical 1.5 tesla MRI scanner is around €1,04 million/US$1.4 million with the lifetime maintenance cost broadly similar to the purchase cost.[109] In the Netherlands, the average MRI scanner costs around €1 million,[110] with a 7T MRI having been taken in use by the UMC Utrecht in December 2007, costing €7 million.[111] Construction of MRI suites could cost up to US$500,000/€370.000 or more, depending on project scope. Pre-polarizing MRI (PMRI) systems using resistive electromagnets have shown promise as a low cost alternative and have specific advantages for joint imaging near metal implants, however they are unlikely to be suitable for routine whole-body or neuroimaging applications.[112][113]

MRI scanners have become significant sources of revenue for healthcare providers in the US. This is because of favorable reimbursement rates from insurers and federal government programs. Insurance reimbursement is provided in two components, an equipment charge for the actual performance and operation of the MRI scan and a professional charge for the radiologist's review of the images and/or data. In the US Northeast, an equipment charge might be $3,500/€2.600 and a professional charge might be $350/€260,[114] although the actual fees received by the equipment owner and interpreting physician are often significantly less and depend on the rates negotiated with insurance companies or determined by the Medicare fee schedule. For example, an orthopedic surgery group in Illinois billed a charge of $1,116/€825 for a knee MRI in 2007, but the Medicare reimbursement in 2007 was only $470.91/€350.[115] Many insurance companies require advance approval of an MRI procedure as a condition for coverage.
In the US, the Deficit Reduction Act of 2005 significantly reduced reimbursement rates paid by federal insurance programs for the equipment component of many scans, shifting the economic landscape. Many private insurers have followed suit.[citation needed]
In the United States, an MRI of the brain with and without contrast billed to Medicare Part B entails, on average, a technical payment of US$403/€300 and a separate payment to the radiologist of US$93/€70.[116] In France, the cost of an MRI exam is approximately €150/US$205. This covers three basic scans including one with an intravenous contrast agent as well as a consultation with the technician and a written report to the patient's physician. [citation needed] In Japan, the cost of an MRI examination (excluding the cost of contrast material and films) ranges from US$155/€115 to US$180/€133, with an additional radiologist professional fee of US$17/€12,50.[117] In India, the cost of an MRI examination including the fee for the radiologist's opinion comes to around Rs 3000–4000 (€37-49/US$50–60), excluding the cost of contrast material. In the UK the retail price for an MRI scan privately ranges between £350 and £500 (€440-630).
Overuse
[edit]Medical societies issue guidelines for when physicians should use MRI on patients and recommend against overuse. MRI can detect health problems or confirm a diagnosis, but medical societies often recommend that MRI not be the first procedure for creating a plan to diagnose or manage a patient's complaint. A common case is to use MRI to seek a cause of low back pain; the American College of Physicians, for example, recommends against this procedure as unlikely to result in a positive outcome for the patient.[118][119]
Specialized applications
[edit]Diffusion MRI
[edit]
Diffusion MRI measures the diffusion of water molecules in biological tissues.[120] Clinically, diffusion MRI is useful for the diagnoses of conditions (e.g., stroke) or neurological disorders (e.g., multiple sclerosis), and helps better understand the connectivity of white matter axons in the central nervous system.[121] In an isotropic medium (inside a glass of water for example), water molecules naturally move randomly according to turbulence and Brownian motion. In biological tissues however, where the Reynolds number is low enough for flows to be laminar, the diffusion may be anisotropic. For example, a molecule inside the axon of a neuron has a low probability of crossing the myelin membrane. Therefore the molecule moves principally along the axis of the neural fiber. If it is known that molecules in a particular voxel diffuse principally in one direction, the assumption can be made that the majority of the fibers in this area are parallel to that direction.
The recent development of diffusion tensor imaging (DTI)[122] enables diffusion to be measured in multiple directions and the fractional anisotropy in each direction to be calculated for each voxel. This enables researchers to make brain maps of fiber directions to examine the connectivity of different regions in the brain (using tractography) or to examine areas of neural degeneration and demyelination in diseases like multiple sclerosis.
Another application of diffusion MRI is diffusion-weighted imaging (DWI). Following an ischemic stroke, DWI is highly sensitive to the changes occurring in the lesion.[123] It is speculated that increases in restriction (barriers) to water diffusion, as a result of cytotoxic edema (cellular swelling), is responsible for the increase in signal on a DWI scan. The DWI enhancement appears within 5–10 minutes of the onset of stroke symptoms (as compared to computed tomography, which often does not detect changes of acute infarct for up to 4–6 hours) and remains for up to two weeks. Coupled with imaging of cerebral perfusion, researchers can highlight regions of "perfusion/diffusion mismatch" that may indicate regions capable of salvage by reperfusion therapy.
Like many other specialized applications, this technique is usually coupled with a fast image acquisition sequence, such as echo planar imaging sequence.
Magnetic resonance angiography
[edit]
Magnetic resonance angiography (MRA) generates pictures of the arteries to evaluate them for stenosis (abnormal narrowing) or aneurysms (vessel wall dilatations, at risk of rupture). MRA is often used to evaluate the arteries of the neck and brain, the thoracic and abdominal aorta, the renal arteries, and the legs (called a "run-off"). A variety of techniques can be used to generate the pictures, such as administration of a paramagnetic contrast agent (gadolinium) or using a technique known as "flow-related enhancement" (e.g., 2D and 3D time-of-flight sequences), where most of the signal on an image is due to blood that recently moved into that plane, see also FLASH MRI. Techniques involving phase accumulation (known as phase contrast angiography) can also be used to generate flow velocity maps easily and accurately. Magnetic resonance venography (MRV) is a similar procedure that is used to image veins. In this method, the tissue is now excited inferiorly, while the signal is gathered in the plane immediately superior to the excitation plane—thus imaging the venous blood that recently moved from the excited plane.[124]
Magnetic resonance spectroscopy
[edit]Magnetic resonance spectroscopy (MRS) is used to measure the levels of different metabolites in body tissues. The MR signal produces a spectrum of resonances that corresponds to different molecular arrangements of the isotope being "excited". This signature is used to diagnose certain metabolic disorders, especially those affecting the brain,[125] and to provide information on tumor metabolism.[126]
Magnetic resonance spectroscopic imaging (MRSI) combines both spectroscopic and imaging methods to produce spatially localized spectra from within the sample or patient. The spatial resolution is much lower (limited by the available SNR), but the spectra in each voxel contains information about many metabolites. Because the available signal is used to encode spatial and spectral information, MRSI requires high SNR achievable only at higher field strengths (3 T and above).[citation needed]
Functional MRI
[edit]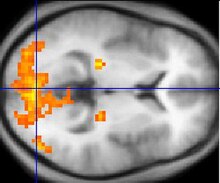
Functional MRI (fMRI) measures signal changes in the brain that are due to changing neural activity. Compared to anatomical T1W imaging, the brain is scanned at lower spatial resolution but at a higher temporal resolution (typically once every 2–3 seconds). Increases in neural activity cause changes in the MR signal via TScript error: No such module "Su". changes;[127] this mechanism is referred to as the BOLD (blood-oxygen-level dependent) effect. Increased neural activity causes an increased demand for oxygen, and the vascular system actually overcompensates for this, increasing the amount of oxygenated hemoglobin relative to deoxygenated hemoglobin. Because deoxygenated hemoglobin attenuates the MR signal, the vascular response leads to a signal increase that is related to the neural activity. The precise nature of the relationship between neural activity and the BOLD signal is a subject of current research. The BOLD effect also allows for the generation of high resolution 3D maps of the venous vasculature within neural tissue.
While BOLD signal analysis is the most common method employed for neuroscience studies in human subjects, the flexible nature of MR imaging provides means to sensitize the signal to other aspects of the blood supply. Alternative techniques employ arterial spin labeling (ASL) or weighting the MRI signal by cerebral blood flow (CBF) and cerebral blood volume (CBV). The CBV method requires injection of a class of MRI contrast agents that are now in human clinical trials. Because this method has been shown to be far more sensitive than the BOLD technique in preclinical studies, it may potentially expand the role of fMRI in clinical applications. The CBF method provides more quantitative information than the BOLD signal, albeit at a significant loss of detection sensitivity.[citation needed]
Real-time MRI
[edit]Real-time MRI refers to the continuous monitoring ("filming") of moving objects in real time. While many different strategies have been developed over the past two decades, a recent development reported a real-time MRI technique based on radial FLASH and iterative reconstruction that yields a temporal resolution of 20 to 30 milliseconds for images with an in-plane resolution of 1.5 to 2.0 mm. The new method promises to add important information about diseases of the joints and the heart. In many cases MRI examinations may become easier and more comfortable for patients.[128]
Interventional MRI
[edit]The lack of harmful effects on the patient and the operator make MRI well-suited for "interventional radiology", where the images produced by an MRI scanner are used to guide minimally invasive procedures. Of course, such procedures must be done without any ferromagnetic instruments.
A specialized growing subset of interventional MRI is that of intraoperative MRI in which the MRI is used in the surgical process. Some specialized MRI systems have been developed that allow imaging concurrent with the surgical procedure. More typical, however, is that the surgical procedure is temporarily interrupted so that MR images can be acquired to verify the success of the procedure or guide subsequent surgical work.[citation needed]
Magnetic resonance guided focused ultrasound
[edit]In MRgFUS therapy, ultrasound beams are focused on a tissue—guided and controlled using MR thermal imaging—and due to the significant energy deposition at the focus, temperature within the tissue rises to more than 65 °C (150 °F), completely destroying it. This technology can achieve precise ablation of diseased tissue. MR imaging provides a three-dimensional view of the target tissue, allowing for precise focusing of ultrasound energy. The MR imaging provides quantitative, real-time, thermal images of the treated area. This allows the physician to ensure that the temperature generated during each cycle of ultrasound energy is sufficient to cause thermal ablation within the desired tissue and if not, to adapt the parameters to ensure effective treatment.[129]
Multinuclear imaging
[edit]Hydrogen is the most frequently imaged nucleus in MRI because it is present in biological tissues in great abundance, and because its high gyromagnetic ratio gives a strong signal. However, any nucleus with a net nuclear spin could potentially be imaged with MRI. Such nuclei include helium-3, lithium-7, carbon-13, fluorine-19, oxygen-17, sodium-23, phosphorus-31 and xenon-129. 23Na and 31P are naturally abundant in the body, so can be imaged directly. Gaseous isotopes such as 3He or 129Xe must be hyperpolarized and then inhaled as their nuclear density is too low to yield a useful signal under normal conditions. 17O and 19F can be administered in sufficient quantities in liquid form (e.g. 17O-water) that hyperpolarization is not a necessity.[citation needed]
Moreover, the nucleus of any atom that has a net nuclear spin and that is bonded to a hydrogen atom could potentially be imaged via heteronuclear magnetization transfer MRI that would image the high-gyromagnetic-ratio hydrogen nucleus instead of the low-gyromagnetic-ratio nucleus that is bonded to the hydrogen atom.[130] In principle, hetereonuclear magnetization transfer MRI could be used to detect the presence or absence of specific chemical bonds.[131][132]
Multinuclear imaging is primarily a research technique at present. However, potential applications include functional imaging and imaging of organs poorly seen on 1H MRI (e.g., lungs and bones) or as alternative contrast agents. Inhaled hyperpolarized 3He can be used to image the distribution of air spaces within the lungs. Injectable solutions containing 13C or stabilized bubbles of hyperpolarized 129Xe have been studied as contrast agents for angiography and perfusion imaging. 31P can potentially provide information on bone density and structure, as well as functional imaging of the brain. Multinuclear imaging holds the potential to chart the distribution of lithium in the human brain, this element finding use as an important drug for those with conditions such as bipolar disorder.[citation needed]
Molecular imaging by MRI
[edit]MRI has the advantages of having very high spatial resolution and is very adept at morphological imaging and functional imaging. MRI does have several disadvantages though. First, MRI has a sensitivity of around 10−3 mol/L to 10−5 mol/L which, compared to other types of imaging, can be very limiting. This problem stems from the fact that the difference between atoms in the high energy state and the low energy state is very small. For example, at 1.5 teslas, a typical field strength for clinical MRI, the difference between high and low energy states is approximately 9 molecules per 2 million. Improvements to increase MR sensitivity include increasing magnetic field strength, and hyperpolarization via optical pumping or dynamic nuclear polarization. There are also a variety of signal amplification schemes based on chemical exchange that increase sensitivity.[citation needed]
To achieve molecular imaging of disease biomarkers using MRI, targeted MRI contrast agents with high specificity and high relaxivity (sensitivity) are required. To date, many studies have been devoted to developing targeted-MRI contrast agents to achieve molecular imaging by MRI. Commonly, peptides, antibodies, or small ligands, and small protein domains, such as HER-2 affibodies, have been applied to achieve targeting. To enhance the sensitivity of the contrast agents, these targeting moieties are usually linked to high payload MRI contrast agents or MRI contrast agents with high relaxivities.[133] A new class of gene targeting MR contrast agents (CA) has been introduced to show gene action of unique mRNA and gene transcription factor proteins.[134][135] This new CA can trace cells with unique mRNA, microRNA and virus; tissue response to inflammation in living brains.[136] The MR reports change in gene expression with positive correlation to TaqMan analysis, optical and electron microscopy.[137]
Other specialized sequences
[edit]New methods and variants of existing methods are often published when they are able to produce better results in specific fields. Examples of these recent improvements are TScript error: No such module "Su".-weighted turbo spin-echo (T2 TSE MRI), double inversion recovery MRI (DIR-MRI) or phase-sensitive inversion recovery MRI (PSIR-MRI), all of them able to improve imaging of brain lesions.[138][139] Another example is MP-RAGE (magnetization-prepared rapid acquisition with gradient echo),[140] which improves images of multiple sclerosis cortical lesions.[141]
Magnetization transfer MRI
[edit]Magnetization transfer (MT) is a technique to enhance image contrast in certain applications of MRI.
Bound protons are associated with proteins and as they have a very short T2 decay they do not normally contribute to image contrast. However, because these protons have a broad resonance peak they can be excited by a radiofrequency pulse that has no effect on free protons. Their excitation increases image contrast by transfer of saturated spins from the bound pool into the free pool, thereby reducing the signal of free water. This homonuclear magnetization transfer provides an indirect measurement of macromolecular content in tissue. Implementation of homonuclear magnetization transfer involves choosing suitable frequency offsets and pulse shapes to saturate the bound spins sufficiently strongly, within the safety limits of specific absorption rate for MRI.[22]
The most common use of this technique is for suppression of background signal in time of flight MR angiography.[142] There are also applications in neuroimaging particularly in the characterization of white matter lesions in multiple sclerosis.[143]
T1rho MRI
[edit]T1ρ (T1rho): Molecules have a kinetic energy that is a function of the temperature and is expressed as translational and rotational motions, and by collisions between molecules. The moving dipoles disturb the magnetic field but are often extremely rapid so that the average effect over a long time-scale may be zero. However, depending on the time-scale, the interactions between the dipoles do not always average away. At the slowest extreme the interaction time is effectively infinite and occurs where there are large, stationary field disturbances (e.g., a metallic implant). In this case the loss of coherence is described as a "static dephasing". T2* is a measure of the loss of coherence in an ensemble of spins that includes all interactions (including static dephasing). T2 is a measure of the loss of coherence that excludes static dephasing, using an RF pulse to reverse the slowest types of dipolar interaction. There is in fact a continuum of interaction time-scales in a given biological sample, and the properties of the refocusing RF pulse can be tuned to refocus more than just static dephasing. In general, the rate of decay of an ensemble of spins is a function of the interaction times and also the power of the RF pulse. This type of decay, occurring under the influence of RF, is known as T1ρ. It is similar to T2 decay but with some slower dipolar interactions refocused, as well as static interactions, hence T1ρ≥T2.[144]
Fluid attenuated inversion recovery (FLAIR)
[edit]Fluid Attenuated Inversion Recovery (FLAIR)[145] is an inversion-recovery pulse sequence used to nullify the signal from fluids. For example, it can be used in brain imaging to suppress cerebrospinal fluid (CSF) so as to bring out periventricular hyperintense lesions, such as multiple sclerosis (MS) plaques. By carefully choosing the inversion time TI (the time between the inversion and excitation pulses), the signal from any particular tissue can be suppressed.
Susceptibility weighted imaging (SWI)
[edit]Susceptibility weighted imaging (SWI), is a new type of contrast in MRI different from spin density, T1, or T2 imaging. This method exploits the susceptibility differences between tissues and uses a fully velocity compensated, three dimensional, RF spoiled, high-resolution, 3D gradient echo scan. This special data acquisition and image processing produces an enhanced contrast magnitude image very sensitive to venous blood, hemorrhage and iron storage. It is used to enhance the detection and diagnosis of tumors, vascular and neurovascular diseases (stroke and hemorrhage), multiple sclerosis,[146] Alzheimer's, and also detects traumatic brain injuries that may not be diagnosed using other methods.[147]
Neuromelanin imaging
[edit]This method exploits the paramagnetic properties of neuromelanin and can be used to visualize the substantia nigra and the locus coeruleus. It is used to detect the atrophy of these nuclei in Parkinson's disease and other parkinsonisms, and also detects signal intensity changes in major depressive disorder and schizophrenia.[148]
See also
[edit]- [[Archivo:
- REDIRECCIÓN Plantilla:Iconos|20px|Ver el portal sobre Medical]] Portal:Medical. Contenido relacionado con Medical.
- Earth's field NMR (EFNMR)
- Electron-spin resonance (spin physics)
- High definition fiber tracking
- History of brain imaging
- Jemris (open source MRI simulator)
- Magnetic immunoassay
- Magnetic particle imaging (MPI)
- Magnetic resonance elastography
- Magnetic Resonance Imaging (journal)
- Magnetic resonance microscopy
- Magnetic resonance (quantum mechanics) for physical & mathematical understanding
- Medical imaging
- Molecular breast imaging[disambiguation needed]
- MRI RF shielding
- Nephrogenic fibrosing dermopathy
- Neuroimaging software
- Nobel Prize controversies
- Nuclear magnetic resonance (NMR)
- Relaxation (NMR)
- Robinson oscillator
- Rabi cycle
- Virtopsy
References
[edit]- ^ "Magnetic Resonance, a critical peer-reviewed introduction". European Magnetic Resonance Forum. Retrieved 17 November 2014.
- ^ Hollingworth W, Todd CJ, Bell MI, Arafat Q, Girling S, Karia KR, Dixon AK (2000). "The diagnostic and therapeutic impact of MRI: an observational multi-centre study". Clin Radiol. 55 (11): 825–31. doi:10.1053/crad.2000.0546. PMID 11069736.
{{cite journal}}
: CS1 maint: multiple names: authors list (link) - ^ a b "iRefer". Royal College of Radiologists. Retrieved 10 November 2013.
- ^ "Manufacturer and User Facility Device Experience". Food and Drug Administration. Retrieved 16 November 2013.
- ^ Wang PI, Chong ST, Kielar AZ, Kelly AM, Knoepp UD, Mazza MB, Goodsitt MM (2012). "Imaging of pregnant and lactating patients: part 1, evidence-based review and recommendations". AJR Am J Roentgenol. 198 (4): 778–84. doi:10.2214/AJR.11.7405. PMID 22451541.
{{cite journal}}
: CS1 maint: multiple names: authors list (link) - ^ Smith-Bindman R, Miglioretti DL, Johnson E, Lee C, Feigelson HS, Flynn M, Greenlee RT, Kruger RL, Hornbrook MC, Roblin D, Solberg LI, Vanneman N, Weinmann S, Williams AE (2012). "Use of diagnostic imaging studies and associated radiation exposure for patients enrolled in large integrated health care systems, 1996-2010". JAMA. 307 (22): 2400–9. doi:10.1001/jama.2012.5960. PMC 3859870. PMID 22692172.
{{cite journal}}
: CS1 maint: multiple names: authors list (link) - ^ "Health at a glance 2009 OECD indicators". OECD. 2009. doi:10.1787/health_glance-2009-en. ISSN 1995-3992.
{{cite journal}}
: Cite journal requires|journal=
(help) - ^ American Society of Neuroradiology (2013). "ACR-ASNR Practice Guideline for the Performance and Interpretation of Magnetic Resonance Imaging (MRI) of the Brain" (PDF).
- ^ Brown RA, Nelson JA (June 2012). "Invention of the N-localizer for stereotactic neurosurgery and its use in the Brown-Roberts-Wells stereotactic frame". Neurosurgery. 70 (2 Supplement Operative): 173–176. doi:10.1227/NEU.0b013e318246a4f7. PMID 22186842.
- ^ Leksell L, Leksell D, Schwebel J (January 1985). "Stereotaxis and nuclear magnetic resonance". Journal of Neurology, Neurosurgery & Psychiatry. 48 (1): 14–18. doi:10.1136/jnnp.48.1.14. PMC 1028176. PMID 3882889.
{{cite journal}}
: CS1 maint: multiple names: authors list (link) - ^ Thomas DG, Davis CH, Ingram S, Olney JS, Bydder GM, Young IR (January 1986). "Stereotaxic biopsy of the brain under MR imaging control". AJNR American Journal of Neuroradiology. 7 (1): 161–163. PMID 3082131.
{{cite journal}}
: CS1 maint: multiple names: authors list (link) - ^ Heilbrun MP, Sunderland PM, McDonald PR, Wells TH Jr, Cosman E, Ganz E (1987). "Brown-Roberts-Wells stereotactic frame modifications to accomplish magnetic resonance imaging guidance in three planes". Applied Neurophysiology. 50 (1–6): 143–152. doi:10.1159/000100700. PMID 3329837.
{{cite journal}}
: CS1 maint: multiple names: authors list (link) - ^ "ACCF/ACR/SCCT/SCMR/ASNC/NASCI/SCAI/SIR 2006 Appropriateness Criteria for Cardiac Computed Tomography and Cardiac Magnetic Resonance Imaging". Journal of the American College of Radiology. 3 (10): 751–771. 2006. doi:10.1016/j.jacr.2006.08.008. ISSN 1546-1440.
- ^ Helms, C (2008). Musculoskeletal MRI. Saunders. ISBN 1416055347.
- ^ Frydrychowicz A, Lubner MG, Brown JJ, Merkle EM, Nagle SK, Rofsky NM, Reeder SB (2012). "Hepatobiliary MR imaging with gadolinium-based contrast agents". J Magn Reson Imaging. 35 (3): 492–511. doi:10.1002/jmri.22833. PMC 3281562. PMID 22334493.
{{cite journal}}
: CS1 maint: multiple names: authors list (link) - ^ Sandrasegaran K, Lin C, Akisik FM, Tann M (2010). "State-of-the-art pancreatic MRI". AJR Am J Roentgenol. 195 (1): 42–53. doi:10.2214/ajr.195.3_supplement.0s42. PMID 20566796.
{{cite journal}}
: CS1 maint: multiple names: authors list (link) - ^ Masselli G, Gualdi G (2012). "MR imaging of the small bowel". Radiology. 264 (2): 333–48. doi:10.1148/radiol.12111658. PMID 22821694.
- ^ Zijta FM, Bipat S, Stoker J (2010). "Magnetic resonance (MR) colonography in the detection of colorectal lesions: a systematic review of prospective studies". Eur Radiol. 20 (5): 1031–46. doi:10.1007/s00330-009-1663-4. PMC 2850516. PMID 19936754.
{{cite journal}}
: CS1 maint: multiple names: authors list (link) - ^ Giussani C, Roux FE, Ojemann J, Sganzerla EP, Pirillo D, Papagno C (2010). "Is preoperative functional magnetic resonance imaging reliable for language areas mapping in brain tumor surgery? Review of language functional magnetic resonance imaging and direct cortical stimulation correlation studies". Neurosurgery. 66 (1): 113–20. doi:10.1227/01.NEU.0000360392.15450.C9. PMID 19935438.
{{cite journal}}
: CS1 maint: multiple names: authors list (link) - ^ Heeger DJ, Ress D (2002). "What does fMRI tell us about neuronal activity?". Nat. Rev. Neurosci. 3 (2): 142–51. doi:10.1038/nrn730. PMID 11836522.
- ^ Husband, J (2008). Recommendations for Cross-Sectional Imaging in Cancer Management: Computed Tomography - CT Magnetic Resonance Imaging - MRI Positron Emission Tomography - PET-CT (PDF). Royal College of Radiologists. ISBN 1 905034 13 X.
- ^ a b McRobbie, Donald W. (2007). MRI from picture to proton. Cambridge, UK ; New York: Cambridge University Press. ISBN 0-521-68384-X.
- ^ Sasaki M, Ehara S, Nakasato T, Tamakawa Y, Kuboya Y, Sugisawa M, Sato T (April 1990). "MR of the shoulder with a 0.2-T permanent-magnet unit". AJR Am J Roentgenol. 154 (4): 777–8. doi:10.2214/ajr.154.4.2107675. PMID 2107675.
{{cite journal}}
: CS1 maint: multiple names: authors list (link) - ^ a b c Lauterbur PC (1973). "Image Formation by Induced Local Interactions: Examples of Employing Nuclear Magnetic Resonance". Nature. 242 (5394): 190–1. Bibcode:1973Natur.242..190L. doi:10.1038/242190a0.
- ^ Rinck PA (2014). "The history of MRI; in: Magnetic Resonance in Medicine, 8th edition; http://magnetic-resonance.org/ch/20-04.html".
{{cite journal}}
: Cite journal requires|journal=
(help); External link in
(help)|title=
- ^ Odeblad E, Lindström G (1955). "Some preliminary observations on the proton magnetic resonance in biological samples". Acta Radiologica. 43: 469–476.
- ^ Carr, Herman (1952). Free Precession Techniques in Nuclear Magnetic Resonance (PhD thesis). Cambridge, MA: Harvard University. OCLC 76980558.[page needed]
- ^ Carr, Herman Y. (July 2004). "Field Gradients in Early MRI". Physics Today. 57 (7). American Institute of Physics: 83. Bibcode:2004PhT....57g..83C. doi:10.1063/1.1784322.
- ^ Encyclopedia of Nuclear Magnetic Resonance. Vol. 1. Hoboken, NJ: Wiley and Sons. 1996. p. 253 http://books.google.com/?id=-OBvMgEACAAJ&dq=Encyclopedia%20of%20Nuclear%20Magnetic%20Resonance.
{{cite encyclopedia}}
: Missing or empty|title=
(help) - ^ MacWilliams B (November 2003). "Russian claims first in magnetic imaging". Nature. 426 (6965): 375. Bibcode:2003Natur.426..375M. doi:10.1038/426375a. PMID 14647349.
- ^ "Best Regards to Alfred Nobel". Retrieved 2009-10-16.
- ^ Damadian R (March 1971). "Tumor detection by nuclear magnetic resonance". Science. 171 (3976): 1151–3. Bibcode:1971Sci...171.1151D. doi:10.1126/science.171.3976.1151. PMID 5544870.
- ^ "The man who did not win". Sydney Morning Herald. 2003-10-17. Retrieved 2007-08-04.
- ^ "Scan and Deliver". Wall Street Journal. 2002-06-14. Retrieved 2007-08-04.
- ^ "Apparatus And Method For Detecting Cancer In Tissue". United States Patent and Trademark Office.
- ^ "NSF history". Sri.com. Retrieved 2011-11-28.
- ^ "Scientist Claims Exclusion From Nobel Prize for MRI". Los Angeles Times. 2003-11-08. Retrieved 2013-02-13.
- ^ "Does Dr. Raymond Damadian Deserve the Nobel Prize for Medicine?". The Armenian Reporter. 2003-11-08. Retrieved 2007-08-05.
- ^ Filler A (October 2009). "Magnetic resonance neurography and diffusion tensor imaging: origins, history, and clinical impact of the first 50,000 cases with an assessment of efficacy and utility in a prospective 5000-patient study group". Neurosurgery. 65 (4 Suppl): A29–43. doi:10.1227/01.NEU.0000351279.78110.00. PMC 2924821. PMID 19927075.
- ^ Lauterbur PC (1974). "Magnetic resonance zeugmatography". Pure and Applied Chemistry. 40: 149–57. doi:10.1351/pac197440010149.
- ^ "First MRI and ultrasound scanning". Benjamin S. Beck.
- ^ "The "Indomitable" MRI". Smithsonian Institution.
- ^ Damadian R, Goldsmith M, Minkoff L (1977). "NMR in cancer: XVI. FONAR image of the live human body". Physiol. Chem. Phys. 9 (1): 97–100, 108. PMID 909957.
{{cite journal}}
: CS1 maint: multiple names: authors list (link) - ^ Hinshaw WS, Bottomley PA, Holland GN (1977). "Radiographic thin-section image of the human wrist by nuclear magnetic resonance". Nature. 270 (5639): 722–3. Bibcode:1977Natur.270..722H. doi:10.1038/270722a0. PMID 593393.
{{cite journal}}
: CS1 maint: multiple names: authors list (link) - ^ University of Aberdeen. "Celebrated scientist donates medal collection".
- ^ "JOHN MALLARD".
- ^ "Science Museum". sciencemuseum.org.uk.
- ^ Sijbers J, Scheunders P, Bonnet N, Van Dyck D, Raman E (1996). "Quantification and improvement of the signal-to-noise ratio in a magnetic resonance image acquisition procedure". Magn Reson Imaging. 14 (10): 1157–63. doi:10.1016/S0730-725X(96)00219-6. PMID 9065906.
{{cite journal}}
: CS1 maint: multiple names: authors list (link) - ^ http://icmic.rad.jhmi.edu/icmicpersonnel/BIOBottomleyP.pdf[dead link][Full citation needed]
- ^ Vaughan T, DelaBarre L, Snyder C, Tian J, Akgun C, Shrivastava D, Liu W, Olson C, Adriany G, Strupp J, Andersen P, Gopinath A, van de Moortele PF, Garwood M, Ugurbil K (December 2006). "9.4T human MRI: preliminary results". Magn Reson Med. 56 (6): 1274–82. doi:10.1002/mrm.21073. PMID 17075852.
{{cite journal}}
: CS1 maint: multiple names: authors list (link) - ^ Qian C, Masad IS, Rosenberg JT, Elumalai M, Brey WW, Grant SC, Gor'kov PL (August 2012). "A volume birdcage coil with an adjustable sliding tuner ring for neuroimaging in high field vertical magnets: ex and in vivo applications at 21.1T". J. Magn. Reson. 221: 110–6. Bibcode:2012JMagR.221..110Q. doi:10.1016/j.jmr.2012.05.016. PMID 22750638.
{{cite journal}}
: CS1 maint: multiple names: authors list (link) - ^ ASTM International (2005). "American Society for Testing and Materials (ASTM) International, Designation: F2503-05. Standard Practice for Marking Medical Devices and Other Items for Safety in the Magnetic Resonance Environment".
{{cite journal}}
: Cite journal requires|journal=
(help) - ^ "Physics of magnetic resonance imaging". My-MS.org. Retrieved 27 April 2012.
- ^
Colletti, P.M., Shinbane, J,S,, Shellock, F. G. (2011). "MR-conditional" pacemakers: the radiologist's role in multidisciplinary management". AJR Am J Roentgenol. 197 (4): 1024. doi:10.2214/AJR.11.7120.
{{cite journal}}
: CS1 maint: multiple names: authors list (link) - ^ "Magnetic resonance safety policy of ucsf". University of California, San Francisco. Retrieved 28 April 2012.
- ^ "Safety in the MR Environment: Ferromagnetic Projectile Objects in the MRI Scanner Room". Pa Patient Saf Advis. 6 (2). Pennsylvania Patient Safety Authority: 56–62. 2009-06. Retrieved 4 February 2015.
{{cite journal}}
: Check date values in:|date=
(help) - ^ Randal C. Archibold, "Hospital Details Failures Leading to M.R.I. Fatality," The New York Times, August 22, 2001
- ^ Donald G. McNeil Jr, "M.R.I.'s Strong Magnets Cited in Accidents," The New York Times, August 19, 2005.
- ^ Hartwig, Valentina; Giovannetti, Giulio; Vanello, Nicola; Lombardi, Massimo; Landini, Luigi; Simi, Silvana (2009). "Biological Effects and Safety in Magnetic Resonance Imaging: A Review". International Journal of Environmental Research and Public Health. 6 (6): 1778–1798. doi:10.3390/ijerph6061778. ISSN 1660-4601.
{{cite journal}}
: CS1 maint: unflagged free DOI (link) - ^ "ACR Guidance Document for Safe MR Practices: 2007". Retrieved 2 August 2010.
- ^ "MRI Design Guide" (PDF). Retrieved 2 August 2010.
- ^ "Committee F04 on Medical and Surgical Materials and Devices." Committee F04
- ^ ASTM Standard F2503 - 13, 2013, "Standard Practice for Marking Medical Devices and Other Items for Safety in the Magnetic Resonance Environment," ASTM International, West Conshohocken, PA, 2003, DOI: 10.1520/C0033-03, Standards F2503.
- ^ Formica D, Silvestri S (April 2004). "Biological effects of exposure to magnetic resonance imaging: an overview". Biomed Eng Online. 3: 11. doi:10.1186/1475-925X-3-11. PMC 419710. PMID 15104797.
{{cite journal}}
: CS1 maint: unflagged free DOI (link) - ^ a b Hartwig, V., Giovannetti, G., Vanello, N., Lombardi, M., Landini, L., and Simi, S. (2009). "Biological Effects and Safety in Magnetic Resonance Imaging: A Review". Int. J. Environ. Res. Public Health. 6 (6): 1778–1798. doi:10.3390/ijerph6061778. PMC 2705217. PMID 19578460.
{{cite journal}}
: CS1 maint: multiple names: authors list (link) CS1 maint: unflagged free DOI (link) - ^ Fiechter M, Stehli J, Fuchs TA, Dougoud S, Gaemperli O, Kaufmann PA. (2013). "Impact of cardiac magnetic resonance imaging on human lymphocyte DNA integrity". European Heart Journal. 34 (30): 2340–5. doi:10.1093/eurheartj/eht184. PMC 3736059. PMID 23793096.
{{cite journal}}
: CS1 maint: multiple names: authors list (link) - ^ Lee JW, Kim MS, Kim YJ, Choi YJ, Lee Y, Chung HW. (2011). "Genotoxic effects of 3 T magnetic resonance imaging in cultured human lymphocytes". Bioelectromagnetics. 32 (7): 535–42. doi:10.1002/bem.20664. PMID 21412810.
{{cite journal}}
: CS1 maint: multiple names: authors list (link) - ^ Simi S, Ballardin M, Casella M, De Marchi D, Hartwig V, Giovannetti G, Vanello N, Gabbriellini S, Landini L, Lombardi M (2008). "Is the genotoxic effect of magnetic resonance negligible? Low persistence of micronucleus frequency in lymphocytes of individuals after cardiac scan". Mutat. Res. 645 (1–2): 39–43. doi:10.1016/j.mrfmmm.2008.08.011. PMID 18804118.
{{cite journal}}
: CS1 maint: multiple names: authors list (link) - ^ Suzuki Y, Ikehata M, Nakamura K, Nishioka M, Asanuma K, Koana T, Shimizu H (2001). "Induction of micronuclei in mice exposed to static magnetic fields" (PDF). Mutagenesis. 16 (6): 499–501. doi:10.1093/mutage/16.6.499. PMID 11682641.
{{cite journal}}
: CS1 maint: multiple names: authors list (link) - ^ a b Knuuti J, Saraste A, Kallio M, Minn H (2013). "Is cardiac magnetic resonance imaging causing DNA damage?". European Heart Journal. 34 (30): 2337–2339. doi:10.1093/eurheartj/eht214. PMID 23821403.
{{cite journal}}
: CS1 maint: multiple names: authors list (link) - ^ Cohen MS, Weisskoff RM, Rzedzian RR, Kantor HL (May 1990). "Sensory stimulation by time-varying magnetic fields". Magn Reson Med. 14 (2): 409–14. doi:10.1002/mrm.1910140226. PMID 2345521.
{{cite journal}}
: CS1 maint: multiple names: authors list (link) - ^ Budinger TF, Fischer H, Hentschel D, Reinfelder HE, Schmitt F (1991). "Physiological effects of fast oscillating magnetic field gradients". J Comput Assist Tomogr. 15 (6): 909–14. doi:10.1097/00004728-199111000-00001. PMID 1939767.
{{cite journal}}
: CS1 maint: multiple names: authors list (link) - ^ Reilly JP (March 1989). "Peripheral nerve stimulation by induced electric currents: exposure to time-varying magnetic fields". Med Biol Eng Comput. 27 (2): 101–10. doi:10.1007/BF02446217. PMID 2689806.
- ^ "The Evolution of Magnetic Resonance Imaging: 3T MRI in Clinical Applications", Terry Duggan-Jahns, www.eradimaging.com
- ^ Price DL, De Wilde JP, Papadaki AM, Curran JS, Kitney RI (February 2001). "Investigation of acoustic noise on 15 MRI scanners from 0.2 T to 3 T". J Magn Reson Imaging. 13 (2): 288–93. doi:10.1002/1522-2586(200102)13:2<288::AID-JMRI1041>3.0.CO;2-P. PMID 11169836.
{{cite journal}}
: CS1 maint: multiple names: authors list (link) - ^ The Open University 2007: Understanding Cardiovascular Diseases, course book for the lesson SK121 Understanding cardiovascular diseases, printed by Cambridge University Press, ISBN 978-0-7492-2677-0 (can be found at OUW), pages 220 and 224.
- ^ "Safety data sheet Nitrogen, refrigerated, liquid" (PDF). BOC. Retrieved 2014-09-11.
- ^ Kanal E, Barkovich AJ, Bell C, Borgstede JP, Bradley WG, Froelich JW, Gilk T, Gimbel JR, Gosbee J, Kuhni-Kaminski E, Lester JW, Nyenhuis J, Parag Y, Schaefer DJ, Sebek-Scoumis EA, Weinreb J, Zaremba LA, Wilcox P, Lucey L, Sass N (2007). "ACR guidance document for safe MR practices: 2007". AJR Am J Roentgenol. 188 (6): 1447–74. doi:10.2214/AJR.06.1616. PMID 17515363.
{{cite journal}}
: CS1 maint: multiple names: authors list (link) - ^ International Electrotechnical Commission 2008: Medical Electrical Equipment – Part 2-33: Particular requirements for basic safety and essential performance of magnetic resonance equipment for medical diagnosis, manufacturers' trade standards [1], published by International Electrotechnical Commission, ISBN 2-8318-9626-6 (can be found for purchase at [2]).
- ^ "Cryogen Awareness Quenching and MRI Safety Training". Falck Productions. Retrieved 10 July 2012.
- ^ "GE Health Care" (PDF). GE. Retrieved 10 July 2012.
- ^ Alorainy IA, Albadr FB, Abujamea AH (2006). "Attitude towards MRI safety during pregnancy". Ann Saudi Med. 26 (4): 306–9. PMID 16885635.
{{cite journal}}
: CS1 maint: multiple names: authors list (link) - ^ Webb JA, Thomsen HS (2013). "Gadolinium contrast media during pregnancy and lactation". Acta Radiol. 54 (6): 599–600. doi:10.1177/0284185113484894. PMID 23966544.
- ^ Hunt CH, Wood CP, Lane JI, Bolster BD, Bernstein MA, Witte RJ (2011). "Wide, short bore magnetic resonance at 1.5 t: reducing the failure rate in claustrophobic patients". Clin Neuroradiol. 21 (3): 141–4. doi:10.1007/s00062-011-0075-4. PMID 21598040.
{{cite journal}}
: CS1 maint: multiple names: authors list (link) - ^ "Siemens Introduces First 1.5 Tesla Open Bore MRI". Medical.siemens.com. 2004-07-29. Retrieved 2010-08-02.
- ^ Tom Kelley and David Kelley (18 October 2013). "Kids Were Terrified of Getting MRIs. Then One Man Figured Out a Better Way". Slate.com.
- ^ "Health at a Glance 2011". OECD. 2011. doi:10.1787/health_glance-2011-en. ISSN 1995-3992.
{{cite journal}}
: Cite journal requires|journal=
(help) - ^ Brenner DJ, Hall EJ (November 2007). "Computed tomography--an increasing source of radiation exposure". N. Engl. J. Med. 357 (22): 2277–84. doi:10.1056/NEJMra072149. PMID 18046031.
- ^ Mathews JD, Forsythe AV, Brady Z, Butler MW, Goergen SK, Byrnes GB, Giles GG, Wallace AB, Anderson PR, Guiver TA, McGale P, Cain TM, Dowty JG, Bickerstaffe AC, Darby SC (2013). "Cancer risk in 680,000 people exposed to computed tomography scans in childhood or adolescence: data linkage study of 11 million Australians". BMJ. 346: f2360. doi:10.1136/bmj.f2360. PMC 3660619. PMID 23694687.
{{cite journal}}
: CS1 maint: multiple names: authors list (link) - ^ Semelka RC, Armao DM, Elias J, Huda W (2007). "Imaging strategies to reduce the risk of radiation in CT studies, including selective substitution with MRI". J Magn Reson Imaging. 25 (5): 900–9. doi:10.1002/jmri.20895. PMID 17457809.
{{cite journal}}
: CS1 maint: multiple names: authors list (link) - ^ Health risks from exposure to low levels of ionizing radiation : BEIR VII Phase. Washington, D.C.: National Academies Press. 2006. ISBN 978-0-309-09156-5.
- ^ Formica D, Silvestri S (2004). "Biological effects of exposure to magnetic resonance imaging: an overview". Biomed Eng Online. 3: 11. doi:10.1186/1475-925X-3-11. PMC 419710. PMID 15104797.
{{cite journal}}
: CS1 maint: unflagged free DOI (link) - ^ Bettmann MA (2004) Frequently asked questions: iodinated contrast agents. Radiographics 24 Suppl 1 ():S3-10. article doi:10.1148/rg.24si045519 PMID 15486247
- ^ "Nephrogenic Systemic Fibrosis" (PDF). ACR Manual on Contrast Material. American College of Radiology. Retrieved 13 October 2012.
- ^ Mak GS, Truong QA (2012). "Cardiac CT: Imaging of and Through Cardiac Devices". Curr Cardiovasc Imaging Rep. 5 (5): 328–336. doi:10.1007/s12410-012-9150-8. PMC 3636997. PMID 23626865.
- ^ "MRI procedure". Royal College of Radiologists. Retrieved 17 November 2013.
- ^ "MRI Design Guide". United States Department of Veterans Affairs. April 2008. Retrieved 12 October 2012.
- ^ "Directive 2013/35/EU of the European Parliament and of the Council. Official Journal of the European Union 2004 L179/1".
- ^ Keevil SF, Lomas DJ (2013). "The European Union physical agents (electromagnetic fields) directive: an update for the MRI community". Br J Radiol. 86 (1032): 20130492. doi:10.1259/bjr.20130492. PMID 24096591.
- ^ "MR Contrast Agents". 2014.
- ^ Murphy KJ, Brunberg JA, Cohan RH (October 1996). "Adverse reactions to gadolinium contrast media: a review of 36 cases". AJR Am J Roentgenol. 167 (4): 847–9. doi:10.2214/ajr.167.4.8819369. PMID 8819369.
{{cite journal}}
: CS1 maint: multiple names: authors list (link) - ^ "ACR guideline". guideline.gov. 2005.
- ^ Thomsen HS, Morcos SK, Dawson P (November 2006). "Is there a causal relation between the administration of gadolinium based contrast media and the development of nephrogenic systemic fibrosis (NSF)?". Clin Radiol. 61 (11): 905–6. doi:10.1016/j.crad.2006.09.003. PMID 17018301.
{{cite journal}}
: CS1 maint: multiple names: authors list (link) - ^ "FDA Drug Safety Communication: New warnings for using gadolinium-based contrast agents in patients with kidney dysfunction". Information on Gadolinium-Based Contrast Agents. U.S. Food and Drug Administration. 23 December 2010. Retrieved 12 March 2011.
- ^ "FDA Public Health Advisory: Gadolinium-containing Contrast Agents for Magnetic Resonance Imaging". fda.gov.
- ^ [3][dead link]
- ^ "ismrm.org MRI Questions and Answers" (PDF). Retrieved 2010-08-02.
- ^ "Response to the FDA's May 23, 2007, Nephrogenic Systemic Fibrosis Update1 — Radiology". Radiology.rsna.org. 2007-09-12. Retrieved 2010-08-02.
- ^ "Managing high value capital equipment in the NHS in England" (PDF). National Audit Office (United Kingdom). Retrieved 30 November 2013.
- ^ "MRI-scanner big financial succes for Philips(Dutch)". Eindhovens Dagblad(ED). Retrieved 18 February 2009.
- ^ "MRI scanner of €7 million in use(Dutch)". Medisch Contact. Retrieved 5 December 2007.
- ^ Morgan P, Conolly S, Scott G, Macovski A (1996). "A readout magnet for prepolarized MRI". Magn Reson Med. 36 (4): 527–36. doi:10.1002/mrm.1910360405. PMID 8892203.
{{cite journal}}
: CS1 maint: multiple names: authors list (link) - ^ Blamire AM (2008). "The technology of MRI--the next 10 years?". Br J Radiol. 81 (968): 601–17. doi:10.1259/bjr/96872829. PMID 18628329.
- ^ Stamford Hospital price quotation October 2008, Stamford CT US
- ^ Goldstein, Wayne M.; Gordon, Alexander C.; Branson, Jill Jasperson; Simmons, Christopher; Berland, Kimberly; Willsey, Daniel S.; Andrews, Amanda L. (March 5–9, 2008). Over-Utilization of MRI in the Osteoarthritis Patient (PDF). Annual Meeting AAOS. San Francisco.
- ^ Current Procedural Terminology code #70553 "2010 Medicare Part B National Summary Data File". Data.gov, An Official Website of the United States Government.
- ^ Ehara S, Nakajima Y, Matsui O (August 2008). "Radiology in Japan in 2008". AJR Am J Roentgenol. 191 (2): 328–9. doi:10.2214/AJR.07.3940. PMID 18647897.
{{cite journal}}
: CS1 maint: multiple names: authors list (link) - ^ Consumer Reports; American College of Physicians. "Five Things Physicians and Patients Should Question" (PDF). Choosing Wisely. presented by ABIM Foundation. Consumer Reports. Retrieved August 14, 2012.
- ^ Consumer Reports; American College of Physicians (April 2012). "Imaging tests for lower-back pain: Why you probably don't need them" (PDF). High Value Care. presented by Annals of Internal Medicine. Consumer Reports. Retrieved August 14, 2012.
- ^ Le Bihan D, Breton E, Lallemand D, Grenier P, Cabanis E, Laval-Jeantet M (November 1986). "MR imaging of intravoxel incoherent motions: application to diffusion and perfusion in neurologic disorders". Radiology. 161 (2): 401–7. doi:10.1148/radiology.161.2.3763909. PMID 3763909.
{{cite journal}}
: CS1 maint: multiple names: authors list (link) - ^ "Diffusion Inaging". Stanford University. Retrieved 28 April 2012.
- ^ Filler A (2009). "The History, Development and Impact of Computed Imaging in Neurological Diagnosis and Neurosurgery: CT, MRI, and DTI". Nature Precedings. doi:10.1038/npre.2009.3267.5.
- ^
Moseley ME, Cohen Y, Mintorovitch J, Chileuitt L, Shimizu H, Kucharczyk J, Wendland MF, Weinstein PR (May 1990). "Early detection of regional cerebral ischemia in cats: comparison of diffusion- and T2-weighted MRI and spectroscopy". Magn Reson Med. 14 (2): 330–46. doi:10.1002/mrm.1910140218. PMID 2345513.
{{cite journal}}
: CS1 maint: multiple names: authors list (link) - ^
Haacke, E Mark; Brown, Robert F; Thompson, Michael; Venkatesan, Ramesh (1999). Magnetic resonance imaging: Physical principles and sequence design. New York: J. Wiley & Sons. ISBN 0-471-35128-8.
{{cite book}}
: CS1 maint: multiple names: authors list (link)[page needed] - ^ Rosen Y, Lenkinski RE (July 2007). "Recent advances in magnetic resonance neurospectroscopy". Neurotherapeutics. 4 (3): 330–45. doi:10.1016/j.nurt.2007.04.009. PMID 17599700.
- ^ Golder W (June 2004). "Magnetic resonance spectroscopy in clinical oncology". Onkologie. 27 (3): 304–9. doi:10.1159/000077983. PMID 15249722.
- ^ Thulborn KR, Waterton JC, Matthews PM, Radda GK (February 1982). "Oxygenation dependence of the transverse relaxation time of water protons in whole blood at high field". Biochim. Biophys. Acta. 714 (2): 265–70. doi:10.1016/0304-4165(82)90333-6. PMID 6275909.
{{cite journal}}
: CS1 maint: multiple names: authors list (link) - ^ I Uyanik, P Lindner, D Shah, N Tsekos I Pavlidis (2013) Applying a Level Set Method for Resolving Physiologic Motions in Free-Breathing and Non-gated Cardiac MRI. FIMH, 2013, [4]
- ^ Cline HE, Schenck JF, Hynynen K, Watkins RD, Souza SP, Jolesz FA (1992). "MR-guided focused ultrasound surgery". J Comput Assist Tomogr. 16 (6): 956–65. doi:10.1097/00004728-199211000-00024. PMID 1430448.
{{cite journal}}
: CS1 maint: multiple names: authors list (link) - ^ Hurd RE, John BK (February 1991). "Gradient-enhanced proton-detected heteronuclear multiple-quantum coherence spectroscopy". Journal of Magnetic Resonance, Series A. 91 (3): 648–653. doi:10.1016/0022-2364(91)90395-a.
- ^ Brown RA, Venters RA, Tang PPPZ, Spicer LD (March 1995). "A test for scalar coupling between heteronuclei using gradient-enhanced proton-detected HMQC spectroscopy". Journal of Magnetic Resonance, Series A. 113 (1): 117–119. doi:10.1006/jmra.1995.1064.
{{cite journal}}
: CS1 maint: multiple names: authors list (link) - ^ Miller AF, Egan LA, Townsend CA (March 1997). "Measurement of the degree of isotopic enrichment of different positions in an antibiotic peptide by NMR" (PDF). Journal of Magnetic Resonance. 125 (1): 120–131. doi:10.1006/jmre.1997.1107.
{{cite journal}}
: CS1 maint: multiple names: authors list (link) - ^ Xue S, Qiao J, Pu F, Cameron M, Yang JJ (2013). "Design of a novel class of protein-based magnetic resonance imaging contrast agents for the molecular imaging of cancer biomarkers". Wiley Interdiscip Rev Nanomed Nanobiotechnol. 5 (2): 163–79. doi:10.1002/wnan.1205. PMID 23335551.
{{cite journal}}
: CS1 maint: multiple names: authors list (link) - ^ Liu CH, Kim YR, Ren JQ, Eichler F, Rosen BR, Liu PK (2007). "Imaging cerebral gene transcripts in live animals". J. Neurosci. 27 (3): 713–22. doi:10.1523/JNEUROSCI.4660-06.2007. PMC 2647966. PMID 17234603.
{{cite journal}}
: CS1 maint: multiple names: authors list (link) - ^ Liu CH, Ren J, Liu CM, Liu PK (2014). "Intracellular gene transcription factor protein-guided MRI by DNA aptamers in vivo". FASEB J. 28 (1): 464–73. doi:10.1096/fj.13-234229. PMID 24115049.
{{cite journal}}
: CS1 maint: multiple names: authors list (link) CS1 maint: unflagged free DOI (link) - ^ Liu CH, You Z, Liu CM, Kim YR, Whalen MJ, Rosen BR, Liu PK (2009). "Diffusion-weighted magnetic resonance imaging reversal by gene knockdown of matrix metalloproteinase-9 activities in live animal brains". J. Neurosci. 29 (11): 3508–17. doi:10.1523/JNEUROSCI.5332-08.2009. PMC 2726707. PMID 19295156.
{{cite journal}}
: CS1 maint: multiple names: authors list (link) - ^ Liu CH, Yang J, Ren JQ, Liu CM, You Z, Liu PK (2013). "MRI reveals differential effects of amphetamine exposure on neuroglia in vivo". FASEB J. 27 (2): 712–24. doi:10.1096/fj.12-220061. PMC 3545538. PMID 23150521.
{{cite journal}}
: CS1 maint: multiple names: authors list (link) CS1 maint: unflagged free DOI (link) - ^ Wattjes MP, Lutterbey GG, Gieseke J, Träber F, Klotz L, Schmidt S, Schild HH (January 2007). "Double inversion recovery brain imaging at 3T: diagnostic value in the detection of multiple sclerosis lesions". AJNR Am J Neuroradiol. 28 (1): 54–9. PMID 17213424.
{{cite journal}}
: CS1 maint: multiple names: authors list (link) - ^ Nelson F, Poonawalla AH, Hou P, Huang F, Wolinsky JS, Narayana PA (October 2007). "Improved identification of intracortical lesions in multiple sclerosis with phase-sensitive inversion recovery in combination with fast double inversion recovery MR imaging". AJNR Am J Neuroradiol. 28 (9): 1645–9. doi:10.3174/ajnr.A0645. PMID 17885241.
{{cite journal}}
: CS1 maint: multiple names: authors list (link) - ^ Nelson F, Poonawalla A, Hou P, Wolinsky JS, Narayana PA (November 2008). "3D MPRAGE improves classification of cortical lesions in multiple sclerosis". Mult. Scler. 14 (9): 1214–9. doi:10.1177/1352458508094644. PMC 2650249. PMID 18952832.
{{cite journal}}
: CS1 maint: multiple names: authors list (link) - ^ Brant-Zawadzki M, Gillan GD, Nitz WR (March 1992). "MP RAGE: a three-dimensional, T1-weighted, gradient-echo sequence--initial experience in the brain". Radiology. 182 (3): 769–75. doi:10.1148/radiology.182.3.1535892. PMID 1535892.
{{cite journal}}
: CS1 maint: multiple names: authors list (link) - ^ Wheaton AJ, Miyazaki M (2012). "Non-contrast enhanced MR angiography: physical principles". J Magn Reson Imaging. 36 (2): 286–304. doi:10.1002/jmri.23641. PMID 22807222.
- ^ Filippi M, Rocca MA, De Stefano N, Enzinger C, Fisher E, Horsfield MA, Inglese M, Pelletier D, Comi G (2011). "Magnetic Resonance Techniques in Multiple Sclerosis". Archives of Neurology. 68 (12): 1514–20. doi:10.1001/archneurol.2011.914. ISSN 0003-9942. PMID 22159052.
{{cite journal}}
: CS1 maint: multiple names: authors list (link) - ^ Borthakur A, Mellon E, Niyogi S, Witschey W, Kneeland JB, Reddy R (November 2006). "Sodium and T1rho MRI for molecular and diagnostic imaging of articular cartilage". NMR Biomed. 19 (7): 781–821. doi:10.1002/nbm.1102. PMC 2896046. PMID 17075961.
{{cite journal}}
: CS1 maint: multiple names: authors list (link) - ^ De Coene B, Hajnal JV, Gatehouse P, Longmore DB, White SJ, Oatridge A, Pennock JM, Young IR, Bydder GM (1992). "MR of the brain using fluid-attenuated inversion recovery (FLAIR) pulse sequences". AJNR Am J Neuroradiol. 13 (6): 1555–64. PMID 1332459.
{{cite journal}}
: CS1 maint: multiple names: authors list (link) - ^ Wiggermann C, Hernandez-Torres E, Vavasour I, Moore W, Laule C, MacKay A, Li D, Traboulsee A, Rauscher A (June 2013). "Magnetic resonance frequency shifts during acute MS lesion formation". Neurology. 81 (3): 211–218. doi:10.1212/WNL.0b013e31829bfd63. PMID 23761621.
{{cite journal}}
: CS1 maint: multiple names: authors list (link) - ^ Reichenbach JR, Venkatesan R, Schillinger DJ, Kido DK, Haacke EM (July 1997). "Small vessels in the human brain: MR venography with deoxyhemoglobin as an intrinsic contrast agent". Radiology. 204 (1): 272–7. PMID 9205259.
{{cite journal}}
: CS1 maint: multiple names: authors list (link) - ^ Sasaki M, Shibata E, Tohyama K, Takahashi J, Otsuka K, Tsuchiya K, Takahashi S, Ehara S, Terayama Y, Sakai A (July 2006). "Neuromelanin magnetic resonance imaging of locus ceruleus and substantia nigra in Parkinson's disease". NeuroReport. 17 (11): 1215–8. doi:10.1097/01.wnr.0000227984.84927.a7. PMID 16837857.
{{cite journal}}
: CS1 maint: multiple names: authors list (link)
Further reading
[edit]- TRTF/EMRF: The history of MRI (Peter A. Rinck, ed). url = http://www.magnetic-resonance.org/ch/20-01.html
- Guadalupe Portal; Aliosvi Rodriguez Whole body magnetic resonance imaging in early diagnosis in Trinidad BMJ (2010) ISSN 1756-1833 url = http://www.bmj.com/rapid-response/2011/12/19/re-whole-body-magnetic-resonance-imaging
- Simon, Merrill; Mattson, James S (1996). The pioneers of NMR and magnetic resonance in medicine: The story of MRI. Ramat Gan, Israel: Bar-Ilan University Press. ISBN 0-9619243-1-4.
{{cite book}}
: CS1 maint: multiple names: authors list (link) - Used MRI Machines and other devices
- Haacke, E Mark; Brown, Robert F; Thompson, Michael; Venkatesan, Ramesh (1999). Magnetic resonance imaging: Physical principles and sequence design. New York: J. Wiley & Sons. ISBN 0-471-35128-8.
{{cite book}}
: CS1 maint: multiple names: authors list (link) - Lee SC, Kim K, Kim J, Lee S, Han Yi J, Kim SW, Ha KS, Cheong C (June 2001). "One micrometer resolution NMR microscopy". J. Magn. Reson. 150 (2): 207–13. Bibcode:2001JMagR.150..207L. doi:10.1006/jmre.2001.2319. PMID 11384182.
{{cite journal}}
: CS1 maint: multiple names: authors list (link)
External links
[edit]- MRI: A Peer-Reviewed, Critical Introduction. European Magnetic Resonance Forum (EMRF)/The Round Table Foundation (TRTF); Peter A. Rinck (editor)
- A Guided Tour of MRI: An introduction for laypeople National High Magnetic Field Laboratory
- The Basics of MRI. Underlying physics and technical aspects.
- Video: What to Expect During Your MRI Exam from the Institute for Magnetic Resonance Safety, Education, and Research (IMRSER)
- International Society for Magnetic Resonance in Medicine
- Srinivas M, Heerschap A, Ahrens ET, Figdor CG, de Vries IJ (July 2010). "(19)F MRI for quantitative in vivo cell tracking". Trends Biotechnol. 28 (7): 363–70. doi:10.1016/j.tibtech.2010.04.002. PMC 2902646. PMID 20427096.
{{cite journal}}
: CS1 maint: multiple names: authors list (link) - Blue Plaque commemorating the manufacture of the first commercial MRI whole body scanner at Osney Mead, Oxford
- Royal Institution Lecture – MRI: A Window on the Human Body
- Animal Imaging Database (AIDB)
- How MRI works explained simply using diagrams
- ASTM International
- U.S. Food & Drug Administration
- Pages with non-numeric formatnum arguments
- Pages with script errors
- Pages using the JsonConfig extension
- Pages using duplicate arguments in template calls
- CS1 maint: multiple names: authors list
- CS1 errors: missing periodical
- CS1 errors: external links
- Wikipedia articles needing page number citations from July 2013
- Articles with invalid date parameter in template
- CS1 errors: missing title
- CS1 errors: bare URL
- All articles with dead external links
- Articles with dead external links from July 2013
- CS1 errors: dates
- CS1 maint: unflagged free DOI
- Articles with dead external links from August 2010
- Articles with hatnote templates targeting a nonexistent page
- Missing redirects
- Wikipedia articles needing style editing from February 2015
- All articles needing style editing
- All articles with unsourced statements
- Articles with unsourced statements from July 2013
- Articles with unsourced statements from November 2008
- Articles with unsourced statements from November 2011
- Articles with unsourced statements from December 2010
- All articles with links needing disambiguation
- Articles with links needing disambiguation from September 2013
- American inventions
- Discovery and invention controversies
- 1973 introductions
- Magnetic resonance imaging
- Articles containing video clips